Advancements in shale reservoir characterization optimize capital expenditures
The continuous evolution of the unconventional resources market in the U.S. has led to the development of technology specifically for shale reservoir characterization. This, in turn, fine-tunes operations during the completion and production phases while optimizing the total capital expenditure of the project.
Unconventional reservoirs require technologies typically not used to produce conventional oil and gas reservoirs. The combination of extensive horizontal wells and hydraulic fracturing has been critical for the success of the shale industry. Hydraulic fracturing and multi-stage completions along horizontal wells are used widely to improve hydrocarbon productivity from permeability-challenged formations.
During a typical hydraulic fracturing operation, frac fluid is injected into a wellbore and penetrates a target formation above the fracture pressure. Depending on the completion and pumping strategy, single or multiple tensile fractures can be initiated from the wellbore wall and then extended into the target layers. The U.S. E&P industry has demonstrated, with great success, how hydraulic fracturing technology can extract economic production from low-permeability reservoirs. However, the optimization of practices, according to the characteristics of each formation, is required to enhance final hydrocarbon recovery.
The induced-fracture characteristics (Conductive Reservoir Volume–CRV) dictate the effectiveness of a hydraulic fracturing operation. Hydraulic fractures often develop irregular and complex geometries, depending on fracture potential, which is a complex function of both geomechanical and petrophysical attributes (Hryb et al., 2014; Meija et al., 2015; Shahri et al., 2015). In fact, the developed fracture geometry and pattern could vary significantly along a horizontal well. Its development within the reservoir is governed by the in-situ state of stresses and geomechanical properties, which could cause unexpected fracture interference within the same well and also communication among the wells in the same pad (Huang et al., 2015; Reza et al., 2017).
Cost-effective production uplift from unconventional reservoirs relies on the creation of new, open surface area, where hydraulic fractures are extended into, and produce from, undepleted zones. However, field observations indicate that infill child well fractures could propagate toward nearby producers and depleted areas, causing unfavored interference, commonly known as frac hits.
A frac hit is a well-to-well communication event, where an offset well, often termed “parent well,” is affected by the hydraulic fracturing treatment in a new in-fill well, called the “child well.” In today’s U.S. field development, frac hits have become a common occurrence because of the tight well-to-well spacing resulting from the drilling of child wells in-between existing producers. As the name suggests, frac hits can be a violent affair, as they are known to be strong enough to damage production tubing, casing, and even wellheads.
These unexpected instances of interference between neighboring fractures could also challenge the fluid and proppant placement for child wells. Considering the associated remediation efforts and/or productivity decline in the existing producers, they become expensive problems and have raised attention in recent operations (Lawal et al., 2013). Therefore, there is a need to effectively fracture wells, without interfering with the existing producers, while reaching unstimulated areas of the reservoir. To achieve this goal, in-depth characterization of the geomechanical conditions around both the child and parent wells is highly demanded (Reza et al., 2017; Shahri et al., 2017; Huang et al., 2018).
A systematic study of depletion effects and the key parameters that control fracture curving allows for improving the infill well fracture design by minimizing the communication between wells while maximizing the hydraulic fracture extent (Reza et al., 2017). Reservoir depletion perturbs the in-situ stress state in the formation around fractured horizontal wells (Guo et al., 2017). Therefore, in a comprehensive simulation to fully investigate frac hit phenomenon, integrating the perturbed stress field (a combined function of stress/formation anisotropy, fluid mobility, pore pressure and operating bottom-hole pressure) is essential (Reza et al., 2017; Zhang and Mark, 2017).
A fracture propagation model, coupled with the altered in-situ stress field, can predict the hydraulic fracture propagation path(s) and their radius of curvature (i.e., if the stress state dictates that the fractures should curve in certain scenarios). The analyses, representing various infill well treatment schedule(s) and uncertainties in reservoir characterizations in a shale formation, will provide the most likely fracture network configuration and potential “what if” scenarios. The resulting infill well fracture geometries can then be imported into a reservoir simulator to quantify and compare the production efficiency, and to identify the optimum design parameters to minimize a frac hit.
Potential use of particulate diverters to manipulate the fracture propagation and reduce the risk of a frac hit also can be simulated and recommended for field operations (Shahri et al., 2017; Huang et al., 2018). Experience shows that infill well fractures between tightly spaced horizontal wells can be curved intentionally. Unfavored communication between wells and fracture coverage area can be mitigated by adjusting stimulation parameters and using diverters to maximize recovery and production. Reliable reservoir characterizations and forward coupled modeling are useful in guiding when to drill and fracture infill child wells, before the altered in-situ stress state challenges the planned completion and negatively impacts the stimulation outcome.
Current challenges in unconventional reservoirs require characterization of geomechanical and petrophysical properties around the child and parent wells. Critical reservoir characterization is obtained through the development of formation evaluation technology that can achieve three major goals: a) high- quality measurements in extensive and hostile lateral sections; b) optimization of operational and logging time; and C) mitigation of operational risks during the data acquisition. The combination of these three factors, and the value during the optimization of the completions phase, represents the opportunity to improve the overall financial performance of unconventional assets.
LWD ULTRAWAVE FRACTURE CHARACTERIZATION
The reservoir natural fracture network, and its interaction with hydraulic fractures, has been identified as one of the production drivers in many of the shale basins of the U.S. While the effect on production can be positive or negative, and the impact varies from basin to basin, proper characterization of fractures becomes critical in tight reservoirs to quantify fracture porosity and permeability enhancement, and thereby optimize field development.
Resistivity tools have been used widely in wireline logging and logging-while-drilling to acquire high-resolution borehole images. However, the common operational practice of using of oil-based mud in shale wells makes data acquisition difficult to reach the desired image quality, using this type of technology.
The need for high-resolution imaging that is not affected by current drilling practices resulted in the development of a new LWD UltraWave ultrasonic tool for borehole imaging and geometry. The ultrasonic imager consists of acoustics transducers operating at high frequency, acting as a transmitter and receiver to provide 360° measurements of travel time and amplitude around the wellbore, taking advantage of the rotation of the drill string. The travel time measurements are used to provide a high-resolution caliper, and the amplitude is used to detect formation features, such as bedding planes, fractures and borehole breakouts.
The LWD ultrasonic tool was used to acquire high-quality wellbore images while drilling infill wells in a limestone-dominated shale, Fig. 1. These images were used to identify and characterize fractures, just hours after the wells reached total depth (TD), allowing the timely detection of different formation features, such as natural fractures, induced fractures and bedding planes, Fig. 2. Detailed and accurate wellbore fracture characterization enabled the optimization of the hydraulic fracture design and operation, leading to improving the operator’s capital expenditure.
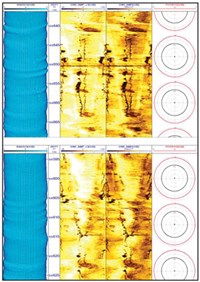
Implementation of the new generation of push-the-bit MAGNUS rotary steerable systems, while acquiring LWD UltraWave ultrasonic imaging, also assured efficient logging operations under common drilling practices. Modern unconventional shale reservoirs are characterized by high-ROP operations, high RPMs, and extensive lateral sections, to achieve fast, economical well construction operations. Then, the application of combined technologies improved drilling operations to ensure not only wellbore integrity, but also reservoir characterization and completions optimization for reservoir productivity enhancement strategies.
CASED HOLE CHARACTERIZATION: BARNETT SHALE
Reservoir characterization through cased hole has multiple advantages. Wireline logging tools are slim, with relatively fast logging speed; are easily deployed in both stable and flowing boreholes, with pre- and post-frac acquisitions; and have multiple logging modes, with data from time and energy spectrums, easily combined with other technologies. A new generation of wireline technologies is designed for simple-to-complex operational challenges.
Raptor 2.0 Pulsed Neutron technology is implemented in cased hole environments to solve reservoir formation challenges by an interpretation mainstream workflow that has four components: 1) data acquisition; 2) evaluation model; 3) consistency; and 4) validation. Cased hole data can be measured, simulated as well as predicted, and they represent the foundation for future validation against each other.
The evaluation model will depend on complexity of formation properties and modeling of the required resolution. Qualitative and quantitative interpretation models are generated to design fit-for-purpose characterization solutions. Consistency component is incorporated with an uncertainty index, which is defined based on consistency of the measurements. Last step is validation, where solutions are cross-validated with the selection of data acquisition and evaluation model. This validation can be internal (measured, simulated and predicted cased hole measurements), as well as external validations with other petrophysics models and measurements.
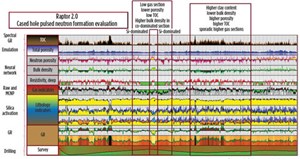
With the logic of mainstream workflow and four signposts, we addressed Barnett shale formation challenges in Texas (Fig. 3) with cased hole pulsed neutron data. Data generated to be analyzed and cross-validated are measured (neutron activation data, as well as elemental counts for main mineralogy components), simulated (total porosity calculated from empirical relationship with core and open-hole data), and predicted (generation of synthetic curves as bulk density, neutron porosity and resistivity). Gas saturation evaluation was provided as a qualitative indicator in this low-porosity reservoir.
However, qualitative interpretation has pre-defined cut-off from relationships between core data and the Monte Carlo N-Particle model. In Fig. 3, gas curve cut-off value represents expected curve response for more than 50% of gas saturation. Consistency was addressed during the data repeatability, as well as correlation with core and cuttings measurements.
Final integrated interpretation was validated with the results from internal data types, models, cores/cuttings, frac results and production. This comprehensive cased-hole shale reservoir solution optimized the completion design (stages and clusters placement) with initial well productivity above forecast.
Advance cased hole characterization in shale wells provides critical reservoir information to optimize completions while ensuring a safer environment, compared with open-hole conditions for data acquisition. The mitigation of operational risks is associated with logging conditions, and the comprehensive and accurate characterization offered by Raptor 2.0 Pulsed Neutron Services.
From reservoir characterization in the shale parent and child wells, a systematic study of depletion effects and the key parameters that control fracture curving allows the improvement of the infill well fracture design by minimizing communication between wells while maximizing the hydraulic fracture extent. Depletion perturbs the in-situ stress tensor in the formation around fractured horizontal wells. Therefore, simulations integrating the perturbed stress field (a function of stress/formation anisotropy, natural and induced fractures, porosity, fluid type, mobility, pore pressure and operating bottom-hole pressure) is essential.
A fracture propagation model, coupled with the altered in-situ stress field, can be utilized to predict the hydraulic fracture propagation path(s) and their radius of curvature (i.e., if the stress state dictates that the fractures should curve). The analyses, performed for different infill well treatment schedule(s), will provide the most likely fracture geometries, considering uncertainties in a shale formation, and potential “what if” scenarios. The resulting infill well fracture geometries can then be imported into a reservoir simulator to quantify the production, and to identify the optimum design parameters to minimize a frac hit. This would include potential use of diverters to influence the fracture propagation and the risk for a frac hit event.
Experience shows that: 1) infill well fractures between tightly spaced horizontal wells can be curved intentionally; and 2) communication between wells and fracture coverage area can be controlled by adjusting stimulation parameters and using diverters to maximize recovery. Forward-coupled modeling can be useful in guiding when to drill and fracture infill wells before the altered stress state negatively impacts production outcome.
Developments of LWD and cased-hole wireline technologies for shale reservoirs are achieved by understanding the reservoir productivity drivers that ultimately impact operational cost during drilling and completions operations, and production performance across the unconventional field. Capital expenditure optimization is achieved by the mitigation of operational NPT, completion design optimization, fracture communication avoidance, and improved hydraulic fracturing strategies.
Operational NPT mitigation during the logging acquisition is obtained by the development of an LWD UltraWave Acoustic Imager that provides high-resolution information while drilling hostile environments, and by the development of the Raptor 2.0 Pulse Neutron Service for a comprehensive unconventional shale characterization in a case-hole environment. Both technologies were used to optimize the completions design through the identification of critical production drivers; this will reveal the strategy to improve CRV for a production enhancement approach.
- Coiled tubing drilling’s role in the energy transition (March 2024)
- Shale technology: Bayesian variable pressure decline-curve analysis for shale gas wells (March 2024)
- What's new in production (February 2024)
- Using data to create new completion efficiencies (February 2024)
- Digital tool kit enhances real-time decision-making to improve drilling efficiency and performance (February 2024)
- E&P outside the U.S. maintains a disciplined pace (February 2024)
- Applying ultra-deep LWD resistivity technology successfully in a SAGD operation (May 2019)
- Adoption of wireless intelligent completions advances (May 2019)
- Majors double down as takeaway crunch eases (April 2019)
- What’s new in well logging and formation evaluation (April 2019)
- Qualification of a 20,000-psi subsea BOP: A collaborative approach (February 2019)
- ConocoPhillips’ Greg Leveille sees rapid trajectory of technical advancement continuing (February 2019)