Understanding the structure of complex flows to optimize production
The oil industry has been going through turmoil over the last five years, and one of the clear outcomes has been to use fewer employees. Much of the human expertise is moving toward retirement, and there is an expectation, through the digitalization process, to use more modeling concepts in the future to improve hydrocarbon production. The second main outcome is to maximize the recovery factor through a better understanding of the reservoir behavior, via topside production optimization.
Behind this vision, it needs to be understood that the automation and transfer of reservoir management to non-humans requires good understanding of multi-phase flows and fluid behaviors, Fig. 1. Indeed, at one stage in the life of a well, it will produce mainly oil, condensate or gas (revenue generators) and water (its disposal costs money). This three-phase flow has seen tremendous modeling improvements and greater understanding over the last two decades, but there is much more to do. Being able to measure flowrates of the three phases in real time is now the main challenge in all types of well conditions.
CHEMICAL REACTION AND FLOW ASSURANCE
Environmental conditions cause additional problems, due to chemical reactions. For example, in water, light hydrocarbons (gas), high pressure and low temperatures create an ideal environment for hydrate, or “burning ice,” formation. Hydrates are a huge safety concern, as they can build up within seconds and obstruct the pipe, potentially halting production or resulting in complete loss of the well.
Oil and gas companies must, therefore, focus first on flow assurance, using expertise and chemicals to mitigate or avoid such a phenomenon, which requires specific knowledge and associated costs. Some of the questions to address are:
- Should we double the (expensive) chemical injection rate to avoid hydrate formation?
- What is the true water flowrate production? Is it completion water, or is it formation water?
These types of issues are becoming a core part of the oil business, especially in deep water offshore, where wellhead pressures are high, the reservoirs are essential light hydrocarbon (gas), and there is a cold environment at seabed (~4°C)—a perfect combination for hydrate formation.
Other chemical reactions also can occur, resulting in less-than-optimal production. For example, by tying back some new, marginal fields to existing production lines, the mixing of different fluids can cause other chemical reactions, causing deposition of wax, asphaltene or other types of solids. Mechanical solutions, like pigging, can be used, but this method increases operational costs and introduces a significant production disruption, if done periodically.
SOLID PRODUCTION AND PIPE INTEGRITY
Additional challenges are associated with pipe integrity. There is a common understanding in the E&P industry that the price of oil and gas will stay within a sustainable range for the coming years. To illustrate, it can be seen that the budget balances for some countries in the Middle East are made with pricing around $70/bbl for the near-future. In addition to the oil and gas prices, themselves, stability of the prices is known to be an important production planning factor. However, the ongoing shale revolution in the U.S. has required OPEC and Russia to make drastic cuts to maintain prices at a reasonable level. One of the consequences of this shale boom is that Opex is limited, and it is also known that natural production will decline with time.
Consequently, to ensure a good return on investment, there is often a demand to escalate production by increasing the well’s choke size. However, this leads to a significantly larger drawdown at the reservoir level and, over time, this may cause reservoir weakening near the wellbore and the resulting production of solids. Also, the maximum critical flow (MCF) before sand production will change over time, due to reservoir pressure change, production of more water, etc., at which point solids could be unexpectedly produced. If not detected at the earliest stages, they will cause erosion.
Additionally, during front-end engineering design (FEED), the pipe design, new production plans, and the way to handle solids may not have been considered, due to the value of gas or oil at the time of the design. Five to ten years later, the production team could be facing some challenging solids production situations. This illustrates the criticality of properly understanding sand management (type, size, fluid velocity, concentration). Sand management also becomes much more complex in remote situations or at the seabed level.
ADDRESSING THE SOLIDS PROBLEM
Failing to maintain and guarantee pipe integrity could create an oil spill that would be catastrophic for the entire industry. While pipe integrity can be addressed by erosion modeling processes, it is not the only parameter to consider—the pressure effect is also important. Indeed, while erosion modeling or erosion modeling standards indicate the possible erosion rates, they can be very optimistic. What happens, in reality, is that the wall thickness of the pipe is gradually reduced by erosion. The instant that the material strength of the pipe is not strong enough, a pipe burst is experienced, which can happen well before full erosion of the pipe’s wall thickness.
Using a computational fluid dynamic (CFD) model also will be overly optimistic, if both factors—sand erosion and line pressure—are not considered together. To address this issue, CFD modeling should be combined with finite element analysis (FEA) in real time, which will provide relevant solutions. Obviously, some of these studies should be correlated, verified and supported with laboratory tests, Fig. 2.
Corrosion also has a significant impact on pipe integrity, especially as the offshore business has some old platforms from the 1970s, which remain operational beyond the originally intended life of 30 to 40 years. Their lifespans cannot be extended forever, so corrosion should not be excluded from the analysis process (as outlined above), and it probably should be a subject of study on its own. While very few CFD companies have the capabilities to use the three effects together (erosion, FEA, corrosion), such comprehensive data analysis and modeling could bring significant value to optimizing production capabilities.
There are two main problems with aging pipes. First, if the wrong operating procedure of a valve is used and a phenomenon like liquid hammering results, this could lead to failure of the pipe. Second, the erosion effect has been demonstrated in a corroded pipe to be a determinant factor with more than 30% higher effect than expected.
THE RIGHT FLUID OR PROCESS
At this stage, we need to introduce the other main element that is very often overlooked—proper understanding of fluid properties and the fact that these properties will change over time. In the case of oil wells, we may all think immediately of the effects of viscosity. With time, the well will produce more water and, for oil with long carbon chain components, an emulsion will be created at the inversion point (point where the continuous phase changes from oil to water). The viscosity of the mixture will be ten times, or greater, than the expected oil viscosity for the same temperature, and this will lead to more friction and a significant decrease in oil production.
So how should this be handled? Should we inject a chemical? Which chemical? Or, should we inject water and expect to be in a water-continuous phase (way beyond the inversion point) with the presence of oil droplets, at the cost of losing some pressure but reducing friction? Should we use some other energy system at cost, but be able to raise the line temperature and, therefore, decrease the friction, Fig. 3?
If the fluid properties or fluid behaviors versus pressure and temperature were not defined properly, or the model using the input data is not correct, then the expected production curve and optimization could be wrong. This also could happen with the presence of two fluids, which may have different intrinsic properties (API quality) and could combine to produce an overall lower quality of oil. The overall production optimization is not only determined by the number of barrels produced, but also the quality of the oil.
A good example is in the case of expected H2S, which will reduce the fluid quality; add some challenges for sampling; and, in terms of pipe integrity, introduce H2S corrosion. This, again, will lead to compromised pipe integrity, especially in areas of the pipe where it has been stressed (i.e. weld points).
The production optimization will be efficient, if all of the above can be done under the same conditions as those experienced in the production environment. Test separation procedures do not accurately reflect the well’s behavior under normal production conditions and, at best, they are only done intermittently. Therefore, there is interest within the industry to have a system that is in line with, and capable of, collecting data (at least the liquid rates, water liquid ratio (WLR) and gas, especially if there is some gas lift optimization or ESP or PCP lifting mechanisms).
The solution, with some lifting processes, is to find the maximum liquid flowrate versus gas lift flowrate, or pump rates-per-minute with the minimum WLR, as this will optimize the oil production flowrate. Permanent monitoring also can allow identification of slugging, which can cause operational problems if separation techniques were used.
IMPROVED DATA ANALYSIS
Imagine that there is only one multi-phase flowmeter for ten wells, and we need four hr of recording for each well. In this case, there are only four out of 40 hr that the well is monitored, or 10% of the time. Is this adequate? With 40% of the information, we start to have an idea, but are still unable to determine a clear statement. The occasional monitoring of wells, therefore, yields little information. This lack of information can be understood when the back-allocation factor for a given manifold or field is determined and applied. It is very often in a range of 15% overestimation from well production.
The industry, therefore, needs to move to a proper digital world where, at any time, information is available from a dedicated program, which can make informed decisions, step-by-step, to keep operations at an optimum level for the field. This will compensate for the human knowledge no longer present in the field, and provide improved, safer working conditions for the people still working in this industry.
Multi-phase flowmeters also are a key enabler for optimization and improved production, providing real-time information that could be used by the reservoir department to refine their models. There is not a perfect multi-phase flowmeter on the market that is capable of working in all conditions within the industry, and a proper selection should be done. However, there are experts and companies capable of helping to short list relevant meters for given field conditions.
CONCLUSION
Throughout this review, the goal has been to show that a global approach should be used to optimize production. It is, therefore, best that a one-stop solution provider is considered, which can factor in all of the different impacts, cumulative effects and their contributions to the flow measurement, pipe integrity, chemical reaction and fluid behavior.
However, the most important element to always take into consideration is the confidence level in the validation of the technology used, and the data produced. In this instance, it is meaningless to deliver a confidence level number if there is not an associated uncertainty, and some form of traceability that is aligned to the process and method that has been used to reference it. Without these elements, there is no way to quantify and act accordingly, for either human or automated processes to effectively rank through the different options algorithmically, to select the optimum solution. A more global, or holistic review, approach should, therefore, be used to account for all means of production optimization. WO
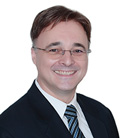
- U.S. footage drilled per well continues to creep higher (October 2024)
- What's new in production: Banking crisis (October 2024)
- First oil: How the U.S. election goes will shape future O&G policy (October 2024)
- Technology advances in casing running extend the reach of automation (October 2024)
- Global activity looks solid and is increasing (September 2024)
- General Bond Log Interpreter completes interpretation, based on standardized rules and data formats (September 2024)