DOE SHASTA initiative aims to improve hydrogen storage
The DOE’s Office of Fossil Energy and Carbon Management (FECM) is leveraging the unique capabilities and demonstrated expertise of four national laboratories—NETL, Pacific Northwest National Laboratory (PNNL), Lawrence Livermore National Laboratory (LLNL) and Sandia National Laboratories (SNL)—to determine the viability, safety and reliability of storing pure hydrogen or hydrogen-natural gas blends in subsurface environments.
Hydrogen (H2 ) is emerging as a low-carbon fuel option for transportation, electricity generation, manufacturing applications and clean energy technologies that will accelerate the United States’ transition to a low-carbon economy. However, a key challenge is to ensure the safe and effective storage of hydrogen. Large-scale storage of H2 can be achieved by utilizing underground resources, similar to how natural gas (NG) has been stored for the past century.
The most cost-effective underground storage option for H2 is depleted hydrocarbon reservoirs, which are reservoirs that do not currently produce oil or gas and are considered to have no economically recoverable hydrocarbons with current technology. They often already have equipment in place for off-take, Fig. 1.
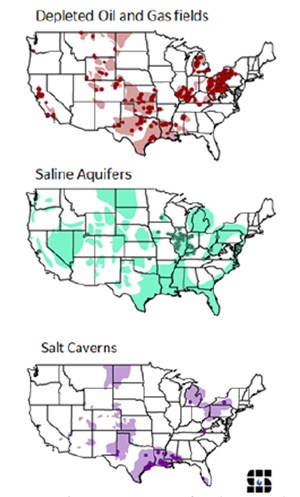
The second most cost-effective underground storage option is aquifers. An aquifer is a body of rock and/or sediment that holds groundwater that can be converted for gas storage. Aquifers are generally close to population centers and consumers. Salt caverns are an attractive option because of lower construction costs, but their capacity is low, compared to reservoirs and aquifers. Hard rock caverns are the most expensive of the storage options.
SHASTA PROJECT
Construction costs of underground storage facilities are generally lower than similar-capacity above-ground facilities. To achieve viable subsurface hydrogen storage, DOE’s FECM announced up to $6.75 million in funding for the Subsurface Hydrogen Assessment, Storage and Technology Acceleration (SHASTA) project It will leverage the capabilities of several national laboratories to determine the viability, safety and reliability of storing pure hydrogen, and associated hydrogen/natural gas blends, in subsurface environments. The SHASTA project will address a number of key issues, including:
- How can the technical and operational risks associated with subsurface hydrogen storage be mitigated, so that operations are protective of humans and the environment?
- How can emerging technologies be leveraged to enable a smart, safe and reliable hydrogen subsurface storage system (e.g., sensors, reservoir simulators and screening tools)?
- What technical, operational and techno-economic insights are needed to enable large-scale subsurface storage for pure hydrogen and handle challenges of hydrogen/natural gas blends in gas storage fields?
ISSUES
Lack of public acceptance. In addition to technical issues discussed below, there is a lack of public acceptance of underground hydrogen storage, says NETL’s Angela Goodman of NETL’s Biogeochemistry and Water Team. “That’s because there is almost no data on public perception of underground hydrogen storage. But there have been studies that focus on hydrogen transportation, above-ground storage, and attitudes about hydrogen as an energy carrier. Those studies generally show a low public awareness of hydrogen as an energy carrier.” Goodman said. SHASTA’s research has indicated that pilot studies, market analyses and lessons from related industries and existing data points can be used to boost public awareness.
“For example, underground storage of natural gas has been well-studied and practiced for more than a century,” Goodman noted. “Geothermal energy projects have dealt with public perception issues, and wind farm projects have faced and often overcome opposition. SHASTA’s team believes that well-communicated results from market analyses and frameworks that assess projects for local populations are key to boosting public acceptance.”
Existing regulations governing the underground storage of natural gas also can provide groundwork for greater public acceptance of underground H2 storage. For example, construction of facilities and pricing practices are often regulated by the Federal Energy Regulatory Commission and state public utility commissions. Safety, meanwhile, has been regulated by a combination of government agencies like the U.S. Department of Transportation’s Pipeline and Hazardous Materials Safety Administration and fire marshals. Environmental regulation is handled by the U.S. Environmental Protection Agency and state departments of environmental protection.
Storage facility integrity. While the four underground storage options above may be viable for underground hydrogen storage, the impacts of underground hydrogen storage on reservoirs, hydrogen leakage risks, and flow behavior of hydrogen and blended mixtures are not well understood. The new demand for widely available hydrogen sources and opportunities to use hydrogen blended with natural gas will require storage reservoirs at locations across the U.S.
“Underground storage of hydrogen is less costly than storage in above-ground vessels,” Goodman noted. “But the technical challenges must be addressed. We are teaming with other national laboratories to focus on quantifying materials compatibility and investigating potential microbial interactions.”
She said NETL researchers are working with colleagues at the Pacific Northwest National Laboratory, the Lawrence Livermore National Laboratory and Sandia National Laboratories under SHASTA to generate knowledge leading to effective, widespread use of underground storage for the nation’s hydrogen and the energy it can bring to bear on decarbonization efforts.
SHASTA researchers are determining the viability, safety and reliability of storing pure hydrogen or hydrogen-natural gas blends in subsurface environments. Goodman notes that experts are supporting the SHASTA initiative by addressing a range of challenges, including:
- Hydrogen storage efficacy for a variety of underground systems, such as depleted hydrocarbon reservoirs, saline aquifers, and salt caverns.
- Effect of hydrogen low-mass density, volumetric energy density and viscosity on gas storage behavior.
- H2 loss through biogeochemical reactions, such as methanogenesis, sulfate reduction and iron reduction.
- Effect of small H2 molecular size on risk of loss of containment from the storage reservoir through caprock, faults or fractures or leaky wells.
- Development of real-time monitoring technology to assess gas migration, potential leakage, microbial activity, and well integrity.
- Support from key stakeholders, as well as public acceptance.
- Delineating expected regulatory environment.
Hydrogen is currently stored in salt caverns in locations around the world, but those geologic formations are not widely available throughout the U.S. The SHASTA work seeks to address that problem by exploring hydrogen storage in saline formations and depleted oil and gas wells that are more geographically disbursed throughout the U.S.
“Our work builds on existing subsurface capabilities that were designed to study wellbore and subsurface reactions,” Goodman said. “The lab’s capabilities will also be used to assess the geochemistry and microbiology of reservoir types targeted for storage and to develop optic fiber sensors needed to monitor underground storage.”
In addition to expert personnel, NETL brings a collection of technical research tools to the SHASTA effort, including equipment that examines wellbore cement integrity, microbial characterization, sensors, testing and many other technical aspects of hydrogen storage. Goodman said the SHASTA initiative “can accelerate and expand the use of hydrogen by using existing natural gas facilities at storage sites across the U.S.; by addressing critical technical hurdles; demonstrating the feasibility of emergent technology; developing tools and technologies to support industry; and by enabling the advancement of subsurface hydrogen storage.”
Wellbore integrity. A wellbore is required at all subsurface hydrogen storage sites to allow injection and removal of the stored hydrogen. During creation of underground storage facilities, a borehole is drilled and lined with steel casing, which is cemented in place. The primary materials of well construction include steel (carbon and/or stainless), polymer elastomers (polymers with elastic properties) and cement. It is important to understand how H2 storage operations can impact the integrity of each of these three materials.
The compatibility of H2 with steel and other metals has been documented in applications like nuclear waste storage. Research also has been conducted that identifies failure mechanisms in elastomers in downhole environments.
NETL has specific expertise in the study of the third material involved in well construction—cement.
Barbara Kutchko, a senior researcher at NETL, who develops advanced cement systems to reduce the environmental footprint of oil and gas drilling operations, is researching how H2 storage operations can impact the integrity of the cement that seals pathways between the surface and underground storage facilities.
With regard to wellbore integrity, “the main challenge for the integrity of wellbore cement is the prevention of gas leakage,” Kutchko said. “Hydrogen is highly mobile in the subsurface and will potentially leak through faulty wells. And hydrogen has the highest diffusion (diffusion is the movement of individual molecules of a substance through a semi-permeable barrier) rate of all gases, because it is the smallest molecule in the universe. That’s why cement integrity must be maintained throughout all phases of underground storage.” “We believe that diffusivity, rather than reactivity in cement will be the challenge to effectively store hydrogen underground,” Kutchko added.
As a primary pathway between the surface and underground storage complex, wells must also endure stresses created by site operations, physical stresses created by injection/withdrawal cycles and constant chemical stresses, all while maintaining structural integrity. Wells that lose control of injected or extracted fluids may become a human health or environmental hazard, if there are leaks into the atmosphere or groundwater in significant quantities.
RESEARCH FOCI
Laboratory work conducted at NETL builds on existing subsurface capabilities designed to study high- pressure, high-temperature reactor studies that simulate wellbore and subsurface reactions, assess the geochemistry and microbiology of reservoir types targeted for H2 / CH4 storage, and develop optic fiber sensors capable of measuring H2, CH4 and pH, Fig. 2. Specific equipment includes the following: gas and ion chromatography, x-ray diffraction; scanning electron microscopy; various mass spectrometry techniques; qPCR and DNA sequencing capabilities; optical fiber sensor design and fabrication; chemical sensor layer synthesis; automated high-temperature high-pressure reactors; Brunauer-EmmettTeller surface area and pore size analysis; TOUGH reservoir simulation; statistical analysis and python coding.
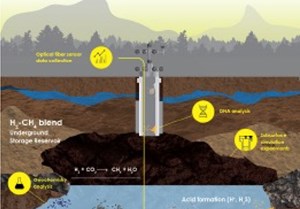
According to Ruishu Wright of NETL’s Functional Materials Team, a range of sensors and wellbore monitoring tools has been used in geological carbon dioxide (CO2) sequestration, the oil and gas industry and other relevant underground storage industry applications. At NETL, a team of experts is helping develop cost-effective approaches for monitoring H2 underground storage facilities over broad areas— capabilities necessary because of the greater mobility and buoyancy of H2 gas plumes. “Surveillance and monitoring are important for evaluating and managing operational risks of underground hydrogen storage,” Wright said. “Therefore, real-time monitoring is needed to assure storage infrastructure integrity and to detect early signs of gas leakage.”
Because industry has used hydrogen in a range of applications for decades, there are many commercial hydrogen sensors in operation, including catalytic combustion sensors, electrochemical sensors, thermo-conductivity sensors, resistive sensors, acoustic leak sensors, and optical based sensors.
“The problem is that existing sensor technologies are mostly point or stand-off hydrogen sensors,” Wright said. “There is a real need for wide-area and long-distance monitoring for hydrogen leak detection in large-scale storage facilities. In addition, the subsurface conditions in some underground storage facilities can be challenging because of higher pressures and temperatures that are not encountered in typical sensor-operating conditions.” That means researchers are at work evaluating emerging sensor technologies, such as optical fiber sensors and passive wireless sensors that are safer in flammable gas mixtures, compared to electrical-based sensors. Researchers are also racing to meet the need for technologies that can monitor groundwater quality to identify geochemical changes that may be required to prevent groundwater contamination. Some geochemical changes can potentially lead to wellbore failures, which are also in need of real-time monitoring.
Another area of focus in H2 storage surveillance and monitoring is induced seismic events: minor quakes and tremors caused by activities like geothermal energy extraction, mining dam building, construction and hydraulic fracturing that alter the stresses and strains on Earth’s crust.
Experience with induced seismic events at underground storage facilities is very limited. But there is significant experience in seismic monitoring and risk management in the oil and natural gas, wastewater disposal, and CO2 sequestration areas that can be applied to seismically problematic gas storage operations. SHASTA efforts are concentrating on making sure all the most useful and effective technologies are brought to bear on the surveillance and monitoring needs of this emerging industry.
RESEARCH PARTNERS
In addition to the work described above to be conducted by NETL, the partnering laboratories have impressive research plans and the equipment to bring those plans to fruition.
Laboratory work conducted at PNNL will leverage capabilities and expertise within the Earth Systems Science Division specifically related to subsurface flow and transport, and biogeochemistry. Specific locations will be the biogeochemistry lab and subsurface science lab. Each of these labs hold the requisite equipment to conduct microbial analysis and flow-through tests. The project also can leverage capabilities available at PNNL’s Environmental Molecular Sciences Laboratory. For computer simulations, PNNL researchers have access to world-class computing facilities, including the Constance system.
Laboratory work conducted at LLNL builds from expertise in rock-water-CO2 interactions in batch and flowing systems. Specific lab locations include the experimental geochemistry facilities equipment necessary for batch exposure experiments. Additional LLNL capabilities co-located in these same buildings include gas and ion chromatography, various mass spectrometry techniques, nuclear magnetic resonance facilities, scanning electron microscopy, X-ray tomography, profilometry, and X-ray diffraction and fluorescence. For computer simulations, LLNL researchers have access to several TOP500 systems through the Livermore Computing Center. Recent studies have concluded that UHS is less costly than storage in above-ground vessels.
Although there is much experience in underground storage of natural gas, there are significant technical challenges that must be addressed to store H2 economically and safely at the commercial scale. “This is a terrific opportunity to lend Sandia’s years of salt cavern storage experience in the Strategic Petroleum Reserve to the challenge of storing hydrogen in geologic systems,” said Don Conley, Sandia manager for geotechnology. “We are pleased to join the SHASTA team and are eager to apply our broader geosciences and system-level expertise to help the project achieve its important goals.”
FACILITIES
The National Energy Technology Laboratory is a U.S. Department of Energy national laboratory that drives innovation and delivers technological solutions for an environmentally sustainable and prosperous energy future. Through its world-class scientists, engineers and research facilities, NETL is ensuring affordable, abundant and reliable energy that drives a robust economy and national security, while developing technologies to manage carbon across the full life cycle, enabling environmental sustainability for all Americans, advancing environmental justice and revitalizing the economies of disadvantaged communities.
The Pacific Northwest National Laboratory advances the frontiers of knowledge, taking on some of the world’s greatest science and technology challenges. Distinctive strengths in chemistry, Earth sciences, biology, and data science are central to our scientific discovery mission. PNNL’s research lays a foundation for innovations that advance sustainable energy through decarbonization and energy storage and enhance national security through nuclear materials and threat analyses.
For more than 60 years, the Lawrence Livermore National Laboratory has applied science and technology to make the world a safer place. Livermore’s defining responsibility is ensuring the safety, security and reliability of the nation’s nuclear deterrent. Yet LLNL’s mission is broader than stockpile stewardship, as dangers ranging from nuclear proliferation and terrorism to energy shortages and climate change threaten national security and global stability. LLNL responds with vision, quality, integrity and technical excellence to the nation’s most challenging security problems.
For more than 70 years, Sandia Labs has delivered essential science and technology to resolve the nation’s most challenging security issues. A strong science, technology and engineering foundation enables Sandia’s mission through a capable research staff working at the forefront of innovation. The laboratories work with other government agencies, industry and academic institutions to accomplish critical missions related to nuclear deterrence, energy technologies and global security. WO